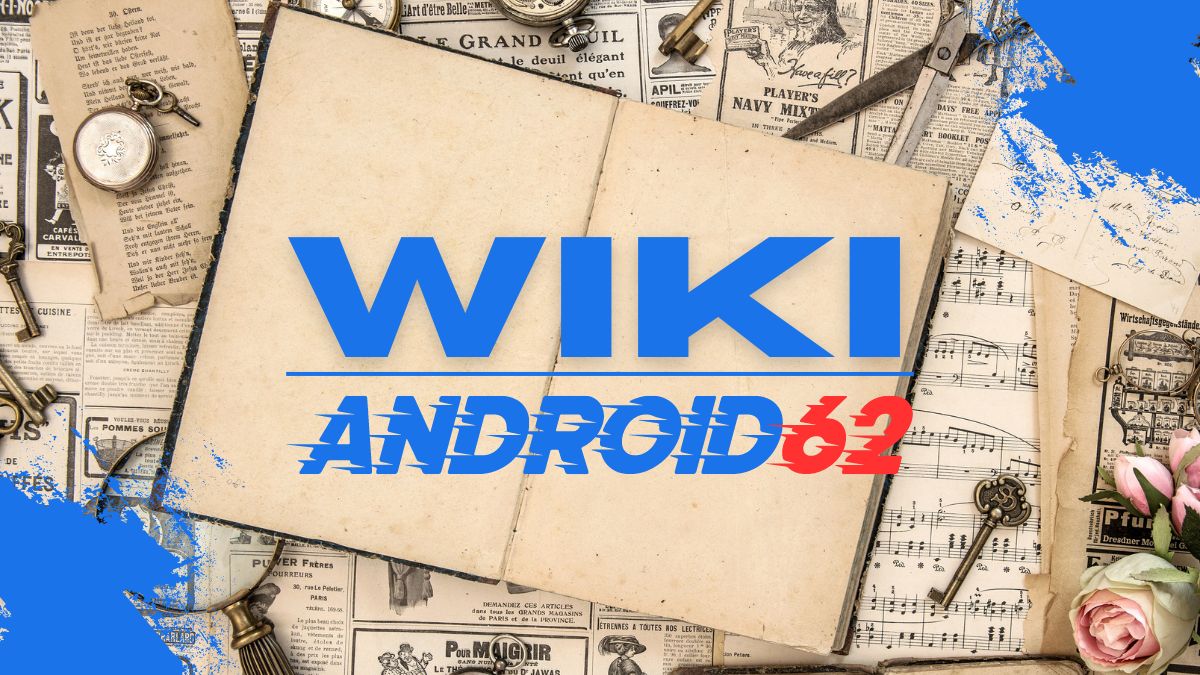
The Role of ATP Synthase in Cellular Energy Production
ATP synthase is a crucial enzyme found in the inner mitochondrial membrane of eukaryotic cells and the plasma membrane of prokaryotic cells. Its main function is to produce adenosine triphosphate (ATP), the primary energy currency in cells, through a process called oxidative phosphorylation. This process relies on the generation of a concentration gradient of protons (H+) across the membrane, which powers ATP synthase to produce ATP. In this article, we will delve into the concentration gradient that powers ATP synthase and its significance in cellular energy production.
The Proton Gradient and ATP Synthase
The proton gradient that powers ATP synthase is established during the electron transport chain (ETC) in cellular respiration. As electrons flow through the ETC, protons are pumped from the mitochondrial matrix (or the cytoplasm in prokaryotic cells) across the inner membrane, creating a higher concentration of protons in the intermembrane space (or periplasmic space in prokaryotic cells) compared to the matrix or cytoplasm. This creates a proton gradient, also known as the proton motive force.
Key points:
– The electron transport chain generates a proton gradient by pumping protons across the inner membrane.
– The proton gradient creates a proton motive force that powers ATP synthase.
Structure and Function of ATP Synthase
ATP synthase is composed of two main subunits: F1 and Fo. The F1 subunit protrudes into the mitochondrial matrix (or the cytoplasm in prokaryotic cells) and houses the catalytic sites responsible for synthesizing ATP. The Fo subunit spans the inner membrane and contains a proton channel through which protons flow back into the matrix (or cytoplasm).
When the protons flow through the Fo subunit, they cause a conformational change in the F1 subunit, which drives the synthesis of ATP from adenosine diphosphate (ADP) and inorganic phosphate (Pi). This process is known as chemiosmotic coupling, where the flow of protons down their electrochemical gradient drives the production of ATP.
Key points:
– ATP synthase is composed of F1 and Fo subunits, with the F1 subunit responsible for ATP synthesis and the Fo subunit for proton translocation.
– The flow of protons through the Fo subunit drives the conformational changes in the F1 subunit that result in ATP synthesis.
The Energy of the Proton Gradient
The proton gradient created by the ETC represents a form of potential energy stored in the form of an electrochemical gradient. This energy is harnessed by ATP synthase to drive the synthesis of ATP. The proton motive force consists of two components: the electrical potential (due to the separation of charge across the membrane) and the chemical potential (due to the difference in proton concentration).
Key points:
– The proton gradient represents potential energy stored in the form of an electrochemical gradient.
– ATP synthase utilizes the energy of the proton motive force to produce ATP from ADP and Pi.
The Importance of ATP Synthase in Cellular Functions
ATP synthase is essential for a wide range of cellular functions. ATP, the product of ATP synthase, serves as the primary energy source for cellular processes such as muscle contraction, nerve impulse transmission, active transport of molecules across membranes, and biosynthetic pathways.
Additionally, ATP synthase plays a crucial role in maintaining cellular homeostasis by regulating the ATP/ADP ratio. This ratio is a key indicator of the energy status of the cell, and ATP synthase helps to balance ATP production with cellular energy demands.
Key points:
– ATP produced by ATP synthase is the primary energy currency in cells and supports various cellular functions.
– ATP synthase helps maintain cellular homeostasis by regulating the ATP/ADP ratio.
Regulation of ATP Synthase Activity
The activity of ATP synthase is tightly regulated to meet the energy demands of the cell and adapt to changing physiological conditions. For example, the proton gradient and the subsequent ATP synthesis are sensitive to the availability of oxygen, as oxygen is the final electron acceptor in the ETC. Changes in pH and mitochondrial membrane potential also influence the activity of ATP synthase.
Furthermore, ATP synthase activity can be modulated by allosteric regulators and post-translational modifications, such as phosphorylation. These regulatory mechanisms allow cells to fine-tune ATP production in response to metabolic demands and environmental cues.
Key points:
– ATP synthase activity is regulated by physiological factors such as oxygen availability, pH, and membrane potential.
– Allosteric regulators and post-translational modifications modulate the activity of ATP synthase.
Implications for Health and Disease
Dysfunction of ATP synthase can have profound implications for human health. Mutations in genes encoding ATP synthase subunits have been linked to various mitochondrial diseases, which can affect tissues with high energy demands, such as the brain, heart, and muscles. These diseases often present with symptoms related to impaired energy production and mitochondrial dysfunction.
Understanding the proton gradient that powers ATP synthase is crucial for developing targeted therapies to address ATP synthase-related disorders. Additionally, research into the regulation of ATP synthase activity may yield insights into metabolic conditions and diseases associated with energy metabolism dysregulation.
Key points:
– ATP synthase dysfunction can lead to mitochondrial diseases and impact tissues with high energy demands.
– Understanding the regulation of ATP synthase activity is important for developing targeted therapies for metabolic disorders.
Conclusion
In summary, the concentration gradient of protons established during cellular respiration plays a central role in powering ATP synthase and the synthesis of ATP. The energy of the proton motive force is harnessed by ATP synthase to drive the phosphorylation of ADP, providing the cell with the energy currency needed for various cellular functions. Understanding the mechanisms and regulation of ATP synthase is not only fundamental to cellular bioenergetics but also holds promise for advancing our knowledge of metabolic diseases and potential therapeutic strategies.